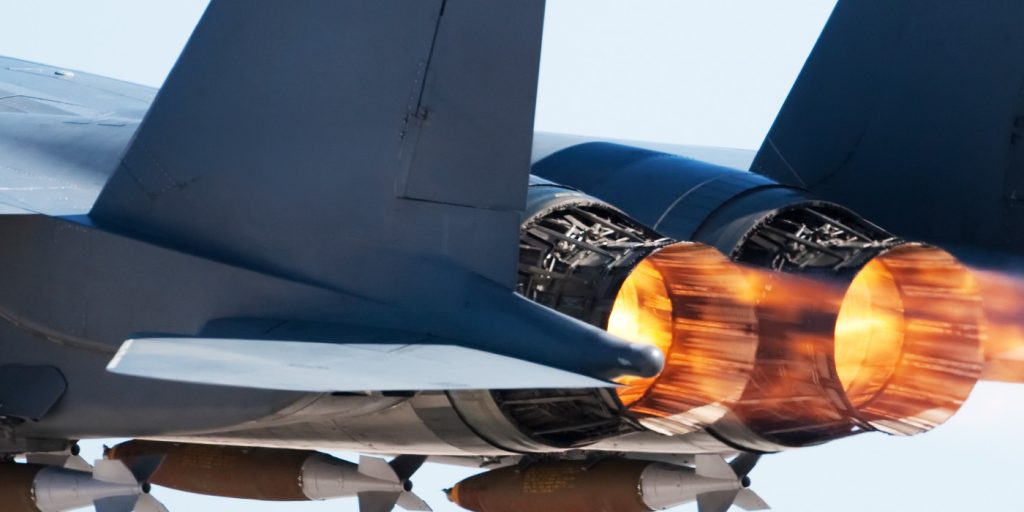
Glass coatings have exhibited remarkable bonding capabilities with various metals and alloys, including stainless steel, titanium, and titanium superalloys, establishing them as effective heat shields capable of safeguarding against corrosion and oxidation at elevated temperatures.1 These attributes have made glass coatings invaluable in the aerospace industry, where aircraft engines must endure highly oxidizing conditions and prolonged exposure to high temperatures. Notably, titanium alloys, renowned for their lightweight and robust properties, exhibit reduced resistance to oxidation and corrosion under such extreme conditions.2 By applying glass coatings to these alloys, their vulnerability to cracks and failure can be mitigated.
Composites of glass and ceramic materials have also proven to be useful coatings.3 The combination of glass and ceramics combines the advantages of glass’ processability with the enhanced mechanical properties that stem from the crystalline nature of ceramics. Tailoring these coatings with a diverse range of components allows for specific thermo-mechanical properties to be achieved, ensuring compatibility with the substrate. An important aspect of their utility lies in the ability to adjust their thermal expansion characteristics to match that of the substrate, making them especially well-suited for high-temperature applications.
This article explores the diverse uses and advantages of glass coatings in heat shielding applications, shedding light on their potential to revolutionize aerospace engineering and other fields reliant on robust thermal protection.
Glass-Ceramic Coatings
Numerous studies have been dedicated to exploring the potential of glass–ceramic coatings for titanium alloys and titanium-aluminum intermetallic compounds.2 These coatings not only offer protection against high-temperature oxidation and corrosion but also exhibit excellent chemical stability and durability.
Glass–ceramic coatings are conventionally prepared using a multi-step melt-quenched method. Raw mineral materials are mixed according to the glass formula and then heated to form a molten glass, usually at temperatures above 1500 °C. The molten glass is quenched in water to produce ‘frit,’ which is then milled into glass powders. These can then be sprayed as a slurry onto a substrate. Finally, the coated component is fired or heat-treated at elevated temperatures. By incorporating ceramic inclusions into the glass matrix and carefully controlling their species and quantity, the thermal and mechanical properties of the glass–ceramic coatings can be customized to meet specific requirements.
In addition, glass composite coatings have demonstrated effective protection for nickel-based superalloys, exhibiting remarkable resistance up to 1000 °C against hot corrosion.4 These superalloys play a crucial role in advanced aircraft, constituting up to half of their total weight. They are prominently featured in numerous rotating turbine components, engine casings, links, and certain engine mounts. Furthermore, these alloys find applications in various other sectors, including turbocharger discs for large diesel engines and high-performance racing car engines.
Recent research has also highlighted the potential of using glass–ceramics in coatings to significantly extend the lifespan of nickel-based superalloys.5 Conventionally, these alloys are coated with a metallic bond coat, followed by a ceramic topcoat. However, at elevated temperatures, oxidation occurs at the bond coat–topcoat interface, leading to the gradual buildup of oxide and inducing stresses within the material, eventually leading to cracks and failure. This oxidation mechanism often determines the lifespan of thermal barrier coatings. Emerging research has also proposed that employing glass–ceramics as the bond coat between the ceramic topcoat and the nickel-based superalloy substrate can effectively prevent this type of oxidation and subsequent degradation. By incorporating glass–ceramics into the coating design, the risk of material failure can be mitigated, considerably extending the overall lifespan of the coating’s thermal barrier.
Space Applications
Glass-based coatings are also proving attractive in one of the harshest environments a vehicle might encounter: space. Using porous silica, NASA scientists developed reaction-cured glass and glass coatings that could be used as coatings for heat shields, as well as for applications such as reaction vessels that need to withstand intense heat and rapid heating and cooling cycles.6
A key example of the significance of these coatings can be observed in NASA’s Space Shuttle Orbiter, the world’s first reusable space vehicle. The success of its missions heavily relied on its heat shield, which had to be able to withstand high temperatures, substantial temperature gradients, and severe thermal shocks during atmospheric re-entry. When the orbiter re-enters the Earth’s atmosphere, the chemical reactions between high-temperature gases and its surface can lead to the vaporization of certain compounds. Hence, any heat shield must endure multiple re-entries into the Earth’s atmosphere at temperatures reaching up to 1400 °C.6
To address these challenges, NASA’s team devised innovative glassy–ceramic metal composites by reacting a combination of glasses, which included a porous high silica borosilicate glass and boron oxide. This mixture was further treated with a selection of additives, such as silicon hexaboride, silicon tetraboride, boron, and boron silicides, to enhance the glass’s stability.
Once applied to an insulation substrate, the NASA composite undergoes several treatments, including glazing in a furnace, resulting in a stable glass with exceptional mechanical properties even at temperatures surpassing 1092 °C.6 These composites prove particularly valuable as coatings for porous silica structures, including reusable surface insulation and foamed silica, operating efficiently in a wide temperature range from -100 °C to 1482 °C.6 Remarkably, they can be utilized at temperatures higher than their glazing temperature, making them a reliable and versatile choice for space applications in the most demanding environments.
Unique Glass Products from Mo-Sci
Mo-Sci specializes in developing custom glass solutions for diverse applications in healthcare, automotive, energy, and the military. Our teams are well-versed in cutting-edge technologies, guiding products from the prototype stage all the way to full-scale commercialization.7
Contact us today to learn more about the solutions on offer.
References and Further Reading
- Chen M. et al. Glass coatings on stainless steels for high-temperature oxidation protection: Mechanisms. Corrosion Science, Volume 82, May 2014, Pages 316-327
- Li W. et al. SiO2–Al2O3–glass composite coating on Ti–6Al–4V alloy: Oxidation and interfacial reaction behavior. Corrosion Science Volume 74, September 2013, Pages 367-378
- Donald I.W et al. Recent developments in the preparation, characterization and applications of glass- and glass–ceramic-to-metal seals and coatings. Journal of Materials Science volume 46, pages1975–2000 (2011)
- Chen M. et al. Preparation and thermal shock behavior at 1000 °C of a glass-alumina-NiCrAlY tri-composite coating on K38G superalloySurface and Coatings Technology Volume 206, Issues 8–9, 15 January 2012, Pages 2566-2571
- Das S. et al. Glass–ceramics as oxidation resistant bond coat in thermal barrier coating system. Ceramics International Volume 35, Issue 4, May 2009, Pages 1403-1406
- Fletcher J.C et al. US Patent. Reaction cured glass and glass coatings. June 6, 1978. Reaction cured glass and glass coatings – NASA Technical Reports Server (NTRS)
- Mo-Sci Corporation. Applications of Thin and Thick Glass Films. 2022. Applications of Thin and Thick Glass Films Mo-Sci Corporation