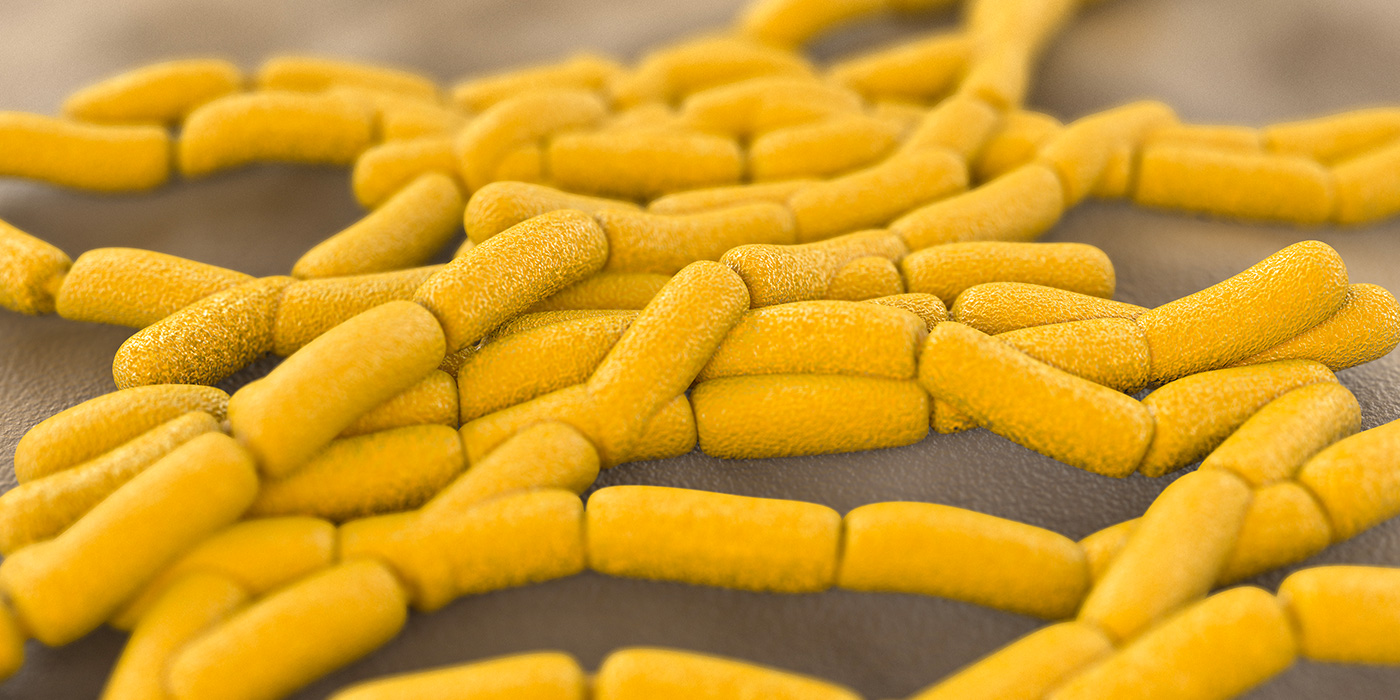
Despite numerous advances in technologies, bacteria remain a cause for concern in both clinical and industrial settings. They pose an especially challenging problem in medicine where only bactericidal strategies which have a minimal detriment to human health are feasible, and bacterial infection continues to be a leading cause of morbidity and mortality.
Surgical procedures in particular are associated with an increased risk of infection, and infection is the most frequently encountered post-surgical complication. The primary means of prevention and treatment of bacterial infections is the administration of antibiotics. However, the power of antibiotics in the fight against infection is diminishing as strains of potent bacteria are developing multi-drug-resistance.
In contrast to historical beliefs, it is now apparent that the majority of bacteria live in surface-bound microbial communities, rather than as free-swimming entities. On binding to the surface, bacteria secrete adhesion proteins that provide an irreversible attachment. The bacteria proceed to proliferate and create a colony that ultimately results in the formation of a mature biofilm protected by a peptidoglycan envelope.1 Such biofilms account for over 80% of clinical microbial infections, and so are associated with considerable morbidity and expense.2
In addition to the possibility of biofilms containing antibiotic-resistant strains, the bacteria are protected from antibacterial agents and the body’s immune system by the peptidoglycan envelope. Biofilm-associated bacteria are 100 to 1,000 times less susceptible to antibiotics than free-swimming bacteria, and so patients with biofilm infections are rarely cured by treatment with antibiotic agents, which are used nonetheless due to the lack of alternatives. Furthermore, there is the scope for bacteria to leave the biofilm and colonize other areas throughout the body.
Consequently, there has been much research in to the development of new broad-spectrum antibiotics and also novel antimicrobial strategies.
Novel antimicrobial materials
Nanotechnology has opened up the potential for the development of new types of materials with antimicrobial properties. It allows the physicochemical properties of a material to be changed in order to achieve antimicrobial effects.3
Antimicrobial nanomaterials include a wide range of metal, metal oxide, and organic nanoparticles and so have numerous modes of action. Although a range of chemical interactions are involved, the end result is membrane damage that leads to loss of integrity and impaired metabolism and ultimately cell death.
The advantage of nanoparticles over antibiotics is that their efficacy depends solely on contact with the bacterial cell wall; they do not need to enter the cell. Consequently, their lethal effect is exerted irrespective of the specific genetics of the target bacteria and is unaffected by the resistance mechanisms used by bacteria to evade antibiotics. Furthermore, the large surface area to size ratio of nanoparticles means that high activity can be achieved with a small dose, whereby minimizing the risk of toxicity. It is therefore hoped that nanoparticles may provide an effective alternative to antibiotics for the treatment of both free-swimming and surface bound bacteria.
Nanoparticles could be used in antimicrobial treatments and in the manufacture of nanocomposite products suitable for use in medical materials and devices.
Bioactive glass
Bioactive glass is a type of glass made from high-purity chemicals, such as silicon oxide, calcium oxide, and phosphorus oxide, that induces specific biological activity.4 Furthermore, by modifying the composition and structure of the glass, its physical properties can be tailored to meet a specific need.4
Bioactive glass elicits a negatively benign immune response and has been widely used in a range of biomedical applications, including tissue engineering, bone grafting, dental reconstruction and wound healing.5 It is able to bond to either hard or soft tissue and has been shown to facilitate strong new bone growth, promote soft tissue regeneration and enhance vascularization to ensure a healthy blood flow to the newly regenerated tissue.6–8 It has also been mixed with dental filling materials to promote the remineralization of dental caries.9
In addition, borate bioactive glass has been shown to have antimicrobial properties against a wide range of bacteria, including MRSA and E-coli.10 It has been shown that bacteria are unable to adhere to bioactive glass and so microfilms cannot develop on its surface.11 This is supported by clinical observations; no infections have been reported on bioactive glass implants.11 In addition, the inclusion of bioactive glass into dental filling material reduced bacterial penetration by 40%, whereby reducing the rate of decay and increasing the lifetime of the restoration.12
The antimicrobial action of bioactive glass can be further increased, giving the glass a broader spectrum of antimicrobial activity, by the addition of ions such as silver, yttrium, selenium, and iodine.13
The use of bioactive glass fibers to promote wound healing and soft tissue repair and the inclusion of bioactive glass in bone grafting and dental restoration composite materials to promote bone growth thus gives dual benefit — more rapid healing and reduced risk of infection.
Mo-Sci produces implant grade bioactive glass powders of varying sizes and with specific compositions suitable for mixing with composite materials.14
References
- Davey ME and O’Toole GA. Microbial biofilms: from ecology to molecular genetics. Microbiology and Molecular Biology Reviews 2000;64(4):847–867.
- Hall-Stoodley L, et al. Bacterial biofilms: from the natural environment to infectious diseases. Nature Reviews Microbiology 2004;2(2): 95–108.
- Karwowska E. Antibacterial potential of nanocomposite-based materials – a short review. Nanotechnology Reviews 2016;6(2):243?254.
- Brauer DS. Bioactive Glasses—Structure and Properties. Angew Chem Int Ed 2015;54: 4160–4181.
- Rahaman MN, et al. Bioactive glass in tissue engineering. Acta Biomaterialia 2011;7:2355?2373.
- Gerhardt L-C and Boccaccini AR. Bioactive Glass and Glass-Ceramic Scaffolds for Bone Tissue Engineering. Materials 2010;3:3867?3910
- Pugely AJ, et al. Influence of 45S5 Bioactive Glass in A Standard Calcium Phosphate Collagen Bone Graft Substitute on the Posterolateral Fusion of Rabbit Spine. Iowa Orthop J. 2017; 37: 193–198.
- Gorustovich A, et al. Effect of bioactive glasses on angiogenesis: In-vitro and in-vivo evidence: A review. Tissue Eng. Part B Rev. 2010;16:199?207.
- Chatzistavrou X, et al. Fabrication and characterization of bioactive and antibacterial composites for dental applications. Acta Biomater. 2014;10:3723–3732. Available at https://www.ncbi.nlm.nih.gov/pubmed/24050766
- Ottomeyer M, et al. Broad-Spectrum Antibacterial Characteristics of Four Novel Borate-Based Bioactive Glasses. Advances in Microbiology 2016;6:776?787.
- Zhang D, et al. Factors Controlling Antibacterial Properties of Bioactive Glasses. Key Engineering Materials 2007;330-332:173?176.
- Khvostenko D, et al. Bioactive glass fillers reduce bacterial penetration into marginal gaps for composite restorations. Dental materials 2016;32(1):73–81. Available at http://www.demajournal.com/article/S0109-5641(15)00437-6/pdf
- Xu Y, et al. Study on the Preparation and Properties of Silver-Doped Borosilicate Antibacterial Glass. Journal of Non-Crystalline Solids 2008;354:1342?1346.
- Mo Sci Corporation website. http://www.mo-sci.com/en/products.