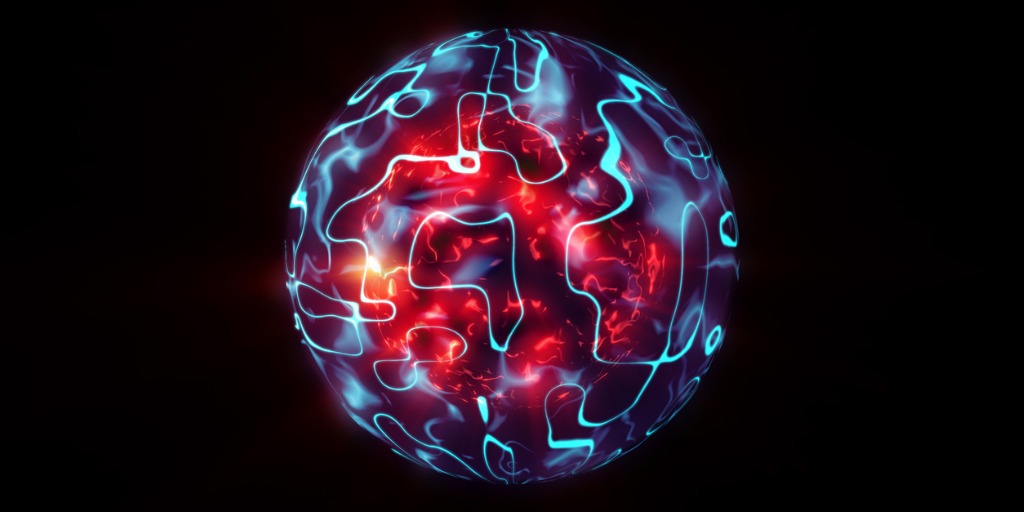
Cold Crucible Induction Melting (CCIM) combines induction heating and water cooling to heat glass melts or metals to very high temperatures while the crucible remains cold. CCIM offers many advantages over conventional melting techniques, including higher process temperatures, the ability to handle corrosive or reactive materials, and the production of extraordinarily pure semiconductors, oxides, and metals.
Basic Principles of Cold Crucible Induction Melting
Initially patented in 1931 by Siemens und Halske Company, Germany; cold crucible induction melting is based on the principles of inductively heating material inside a crucible while the crucible itself is water-cooled.1
A typical CCIM setup consists of a water-cooled steel crucible surrounded by one or more induction coils.2,3 These induction coils carry high-frequency alternating currents, which induce electrical currents in the material being heated. These currents, known as eddy currents, heat the material. Inductive heating of the material is made possible by segmenting the crucible into pieces separated by narrow slits to allow the permeation of magnetic fields.
The crucible is water-cooled — typically, its temperature doesn’t exceed 200°C — causing any glass or metal in contact with the crucible wall to freeze solid. This means that the molten material is fully enclosed within a solid crust or “skull” — earning the process the alternative name of induction skull melting.
CCIM systems are configured so that maximum heating occurs in the middle of the crucible. With maximum power density at the center of the crucible, the extreme temperature gradient between the hot center and the cold walls ensures that the melt is stirred by convective means, as well as by electromagnetic effects.4,5
Advantages of Cold Crucible Induction Melting
In CCIM, the solid crust prevents the molten material from coming into contact with the crucible. This protects the crucible from extreme temperatures and corrosion, giving CCIM several unique advantages.
High Operational Temperatures
Perhaps surprisingly, cooling of the crucible enables higher working temperatures within the melt compared to conventional electric melters. For example, joule-heated melters (JHM) operate by conducting an electric current between electrodes submerged in the molten material. This design is inherently limited by the electrodes’ maximum operational temperature — joule-heat melting is typically limited to applications below 1200°C.6 However, in CCIM, where current is induced rather than conducted, there is no such limitation. Cooling of the crucible further protects CCIM systems from heat damage.
Compatibility with Corrosive Materials
The layer of frozen material between crucible and melt also protects the crucible from corrosion. Separation of the crucible from reactive substances in the melt means that CCIM is compatible with a wider range of materials than other electric melting techniques, including reactive metals such as Zr and Ti.
High Purity Materials
In conventional melting techniques, crucibles are lined with high-performance refractory materials to protect them from heat and corrosion. Though these materials can be effective, very high working temperatures or reactive substances still cause them to degrade, thus contaminating the melt with small amounts of the crucible lining.
As CCIM naturally prevents deterioration of the crucible, the material requirements of CCIM are lower than those of conventional electric melters, as the need for the refractory materials is eliminated.7 Importantly, this means that the melt is not contaminated by the crucible lining, enabling the processing of very high purity materials such as refractory metals and their alloys.
Applications of Cold Crucible Melting
Nuclear Waste Processing
One of the primary applications of CCIM is the vitrification of nuclear waste. In this process, nuclear waste products are mixed with glass-forming chemicals and heated to high temperatures, then formed and solidified into canisters to immobilize the waste in a non-leaching and durable form.
CCIM is particularly well suited to this process as it eliminates the stringent material requirements associated with joule-heating-based vitrification processes. Changing corroded refractories and electrode materials from failed joule heating melters carries a significant radiation exposure risk, which CCIM avoids. In addition, the cold crucible design is smaller, less expensive, and ultimately generates less waste for disposal.2
Production of High Purity Metals and Alloys
The intrinsic corrosion-resistance of CCIM crucibles means that the process is well-suited to the production of high purity refractory metals and alloys, including Titanium, Tantalum, Niobium, and Molybdenum. CCIM can also be used to produce very large pure silicon crystals and massive multi-crystalline silicon ingots as a base material for manufacturing solar cells.
Glass and Oxide Melting
CCIM is an excellent method to melt materials like oxides and silica glass, which are characterized by high melting points and low electrical conductivity at low temperatures. Materials that are insulators at low temperatures (like silica glass) must be pre-heated to a temperature where inductive coupling (i.e., transfer of energy from induction coils to material) can be achieved. Resistance to corrosion means that CCIM techniques can be used to produce high purity glass.8
Phosphate glasses are widely used in optical, biomedical, nuclear, and many other industries. CCIM can also be an alternative method to melt the phosphate glasses that are corrosive to contact refractories and metals at processing temperatures.
Mo-Sci is an expert in glass production and uses various manufacturing processes to produce custom glass solutions at all scales, from prototyping to commercialization. To find out more about our custom melting and glass development services, get in touch today.
References and Further Reading
- Mühlbauer, A. History of Induction Heating and Melting. (Vulkan-Verlag GmbH, 2008).
- Gombert, D. & Richardson, J. G. Cold Crucible Induction Melter Technology: Results of Laboratory Directed Research and Development. INEEL/EXT-01-01213, 910987 http://www.osti.gov/servlets/purl/910987-kFgCJc/ (2001) doi:10.2172/910987.
- Fluxtrol | Modeling and Optimization of Cold Crucible Furnaces for Melting Metals. https://fluxtrol.com/modeling-and-optimization-of-cold-crucible-furnaces-for-melting-metals.
- Pericleous, K., Bojarevics, V., Djambazov, G., Harding, R. A. & Wickins, M. Experimental and numerical study of the cold crucible melting process. Applied Mathematical Modelling 30, 1262–1280 (2006).
- Sombret, C. G. Cold Crucible Melting: A Multipurpose Technique. Proc. Vol. 1994–13, 705–712 (1994).
- Cold Crucible Induction Melting :: Total Materia Article. https://www.totalmateria.com/page.aspx?ID=CheckArticle&site=ktn&LN=ES&NM=389.
- Goyal, P., Verma, V., Singh, R. K. & Vaze, K. K. Thermal Analysis of Joule Heated Ceramic Melter. 4 (2010).
- Chen, R. et al. Glass melting inside electromagnetic cold crucible using induction skull melting technology. Applied Thermal Engineering 121, 146–152 (2017).