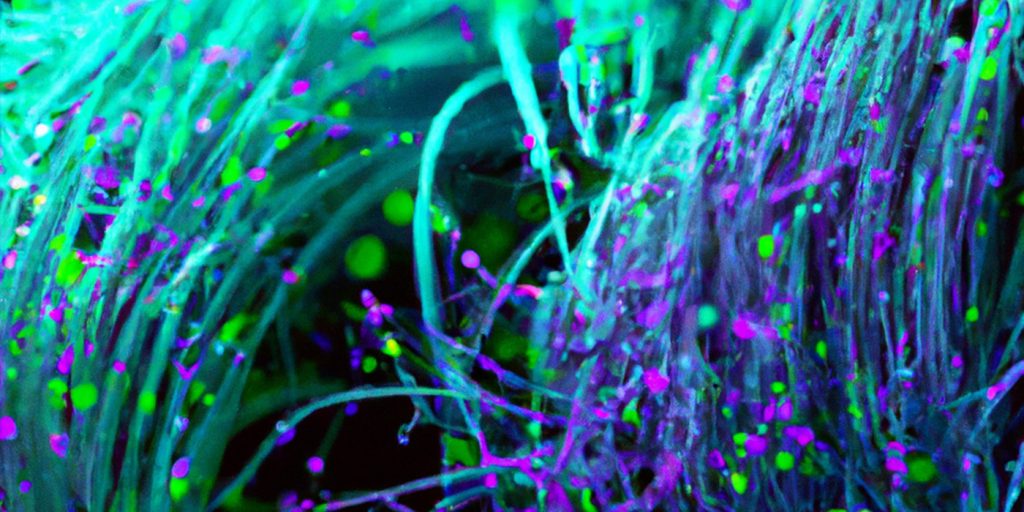
What is Bioactive Glass?
Bioactive glasses (or bioglasses) are silica-based biomaterials that are made up of a three-dimensional silicon-oxide network that includes sodium, calcium, and phosphorus oxides. They are compatible with the human body, bond to bone and soft tissue, and can stimulate new bone growth. This means that such biomaterials have the potential to restore diseased or damaged bone to its original state.1 By adjusting their composition and structure, it is also possible to tailor their physical properties to meet a specific need and control how long they last in the body before they degrade.2
Bioactive Glass History and Development
The first such material was developed in 1969 by Larry Hench, a professor of ceramic engineering at the University of Florida who collaborated with doctors during the Vietnam War to develop a material that could replace bone and other types of tissue in severely wounded soldiers.3 His discovery resulted in a whole new field of research into ‘bioactive’ materials.
At the time, biomaterials research was focused on making materials as unreactive as possible, reducing the body’s inflammatory response and increasing the chances that the body would not reject the implant material or device. Researchers were unaware then that an artificial material had the potential to aid in the healing or regeneration of tissue.
However, what Hench’s team did discover was that their material — later trademarked as Bioglass® — was able to bond with bone. This was the first time scientists had observed an implanted material forming a surface bond with tissue.4 This first bioactive glass, also known as 45S5, was made of four components: silicon dioxide, calcium oxide, sodium oxide, and phosphorus pentoxide. The name 45S5 signifies the 45% weight made up of silicon dioxide and the 5:1 molar ratio of calcium to phosphorus. This composition became critical in making the material highly reactive in aqueous fluids and bioactive.
Hench’s team also tested bone implants of the material in rats and found that they were hard to remove after six weeks. They reported that the strength of the interfacial bond between Bioglass® and bone was equal to, or greater than, the strength of the host bone.5 Over time, it also became clear that Bioglass® could bond with soft connective tissues.6
The researchers investigated a range of compositions. This included experimenting with levels of silica, adding boron and fluoride, and comparing amorphous to crystallized states. Hench found that different compositions affected the rate of bone bonding and the strength and stability of the bond. For 45S5, bonding was fast at silica levels in the range of 42–53%, took 2–4 weeks at 54–60% silica levels, and did not happen at all at more than 60% silica.
The team then went on to work out the sequence of reactions after the bioactive glass had been implanted in bone or soft tissue. This complex, multistage process results in the formation of a biologically active hydroxycarbonate apatite (HCA) layer. Similar to the mineral phase in bone, it appears to help bone cells stick to and grow on the implant surface and is eventually replaced by bone.1
Later, in the 1990s, it was observed that these glasses release biologically active, soluble silica and calcium ions, which stimulate the cells that secrete the material for bone formation (osteoblasts) and stem cells.1 This work by Hench and others demonstrated that ionic release, as well as the HCA layer, was how bioactive glasses stimulate cell growth and accelerate the healing process through rapid tissue repair. It is now known that some bioactive glasses can stimulate the development of new blood vessels (angiogenesis) and have antimicrobial activity thanks to this release of ions.
Overcoming Challenges
Despite its positives, the original bioglasses did have some drawbacks. It tended to be mechanically weak and brittle.7 To make them stronger and more fracture-resistant, scientists designed bioactive glass polymer hybrids.8 In these materials, if the production process is controlled carefully, networks of organic and inorganic components mingle and bond at the nanoscale. The researchers also found that the mechanical properties and degradation rates of hybrids are easier to control than with original bioactive glasses.
It was also challenging to make the original biomaterials into porous structures suitable for scaffolds in tissue engineering. This is because they crystallize during sintering (a heat treatment process used to produce porous materials). Scientists overcame this by making the glass via a different process.8 Traditionally, when making glass (melt-quenching), the process involves temperatures above 1300 °C. However, the sol-gel chemistry-based approach allows nanoparticles of silica to assemble at room temperature. Other advances in processing (foaming, solid freeform fabrication, and nanofiber spinning) mean that porous bioactive glass scaffolds can now be produced from melt and sol-gel glasses.
More recently, advances in nanotechnology have allowed scientists to produce bioactive glass nanoparticles which can be tracked within cells or transport therapeutic or genetic material.8 Both melt and sol-gel glasses can be manufactured as nanoparticles.
Applications of Bioactive Glass
Since its first application as a middle ear prosthesis in 1985, bioactive glass has been widely used in a range of biomedical applications, including bone grafting, dental reconstruction, and wound healing. This versatility stems from the ability to stimulate new bone and soft tissue growth and new blood vessels that provide nutrients to the new tissue.
What is more, these glasses can be formulated differently to suit each application. For example, particulate forms work well as spine implants, fine particulates for dental applications, and fibrous glasses as wound dressings. More recently, the focus has turned to tissue engineering and cell-seeded porous scaffolds that can fully integrate into the body.
Silicate-based bioglasses have also found application in a number of orthopedic and dental applications. Variations on the original material include bioglass 13-93, which contains more silicate (50-56%) than 45S5 and incorporates network modifiers, such as potassium and magnesium oxides. It is just as good as encouraging bone to grow but is stronger than 45S5 and degrades more quickly.9 This material is now used for bone grafts and in the repair of periodontal and facial defects, wound care, and blood loss control.
Some bioglasses are known to possess antibacterial properties, such as S53P4, which contains 53% silica and smaller weights of sodium, calcium, and phosphorus than 45S5. It was developed in the early 1990s at Åbo Akademi University and the University of Turku in Finland.10 When the implant comes into contact with bodily fluids, sodium ions are released, which increases pH; other released ions combine to increase salt concentration levels, pushing up osmotic pressure. These conditions hamper bacterial growth.
The antimicrobial effects of bioactive glass can be boosted by adding ions, such as boron, copper, silver, yttrium, and iodine, or organic nanoparticles. Borate-based glasses have been shown to have antimicrobial properties against a wide range of bacteria, including MRSA and E-coli; as demonstrated by research, bacteria are unable to stick to these glasses, making them particularly useful in preventing infections in wounds and burns.
One such example is Mirragen®, developed by a team at Mo-Sci Corporation (Rolla, Missouri, USA) involving Mo-Sci cofounder Delbert Day and Steven Jung, a graduate student at the Missouri University of Science and Technology and now Chief Technology Officer at Mo-Sci.11 Working with the late Ted Day, former CEO of Mo-Sci, Jung refined the material to gain FDA approval in 2017 to treat wounds. A similar product, Rediheal®, is used by veterinarians to heal major wounds in animals.
The fibrous glass, which has a high calcium content, was the world’s first bioactive glass-based wound care product and has now been shown to aid in the healing of chronic diabetic ulcers. Its fiber structure allows Mirragen® to absorb fluid from the wound site and facilitate healing.
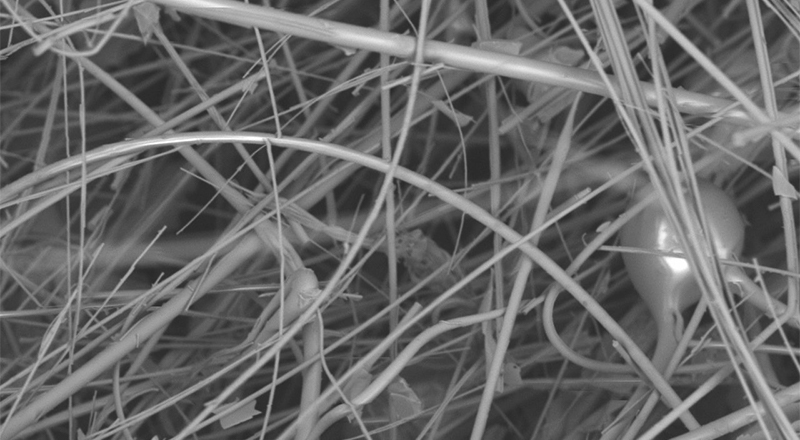
As well as keeping infection at bay, borate-based bioglasses help tissue regenerate by encouraging cells to secrete growth factors involved in the regrowth of blood vessels, which are key for tissue development as they provide all the required nutrients. As such, bioactive glass is highly beneficial when it comes to creating tissue engineering scaffolds, as previously recombinant growth factors were required.
These materials also speed up the healing process as borate-based glasses react five to ten times faster than 45S5 during the conversion process — the process during which a bone graft is converted into hydroxyapatite which can then be remodeled into bone. As a result, borate-based glasses have also been used as coatings for orthopedic and dental implants,12 bone grafts,13 and materials used for tooth fillings.14
Another class of bioglasses is based on phosphates and often contains other additives, such as copper, zinc, or silver oxides. They are less rigid than silicate glasses and can be prepared in different forms, including discs, microspheres, and fibers.15 These glass fibers can also form microtubes, which can be incorporated within various polymers to help nutrients diffuse and blood vessels regrow when used as scaffolds for tissue regeneration. Microspheres have been used in radiotherapy as they provide a stable surface for cells to grow and prevent tissue damage.
Mo-Sci’s Role in Bioglasses
On the market today, most of the bioactive glass that is used in medical devices is manufactured by Mo-Sci,16 and can be traced back to the early days of the first bioglasses when Larry Hench chose the young company to make the glass he needed to manufacture products through his company U.S. Bioglass.
Mo-Sci was founded in 1985 by Delbert Day as a spin-off from his work at the Missouri University of Science and Technology on radioactive glass spheres. The company’s first product was a glass microsphere used in TheraSphere®, a technology designed to deliver tiny amounts of radioactive materials to treat inoperable liver cancer.
Since then, Mo-Sci has developed an extensive range of bioactive glasses. These include microspheres, porous structures, and glass powders (frit). These materials are available in different compositions and sizes depending on the form of the glass and the intended application. Mo-Sci also facilitates custom-made bioactive glass for specific strength and degradation rate requirements.
Mo-Sci’s products are suitable for use in a wide variety of medical and healthcare applications, from coatings for implants to powders for mixing with composite materials to tissue engineering scaffolds. Mo-Sci’s newest bioactive glasses are borate-based, using boron instead of silica as the glass former (see Mirragen® above), and the company has an exclusive license for both hard and soft tissue applications for these glasses. Engineered Tissue Solutions (ETS), a Mo-Sci spin-off company, now distributes Mirragen®.
The company works closely with the healthcare industry and medical professions. For example, it designed the highly porous material Porogran in collaboration with surgeons for use in implantable scaffolds and grafts. Porogran is suitable for use as a granular material or processed into putty or collagen strips. Due to its high surface area, reactions happen more quickly. Porogran can be manufactured in several bioactive glass compositions, such as 45S5 and S53P4.
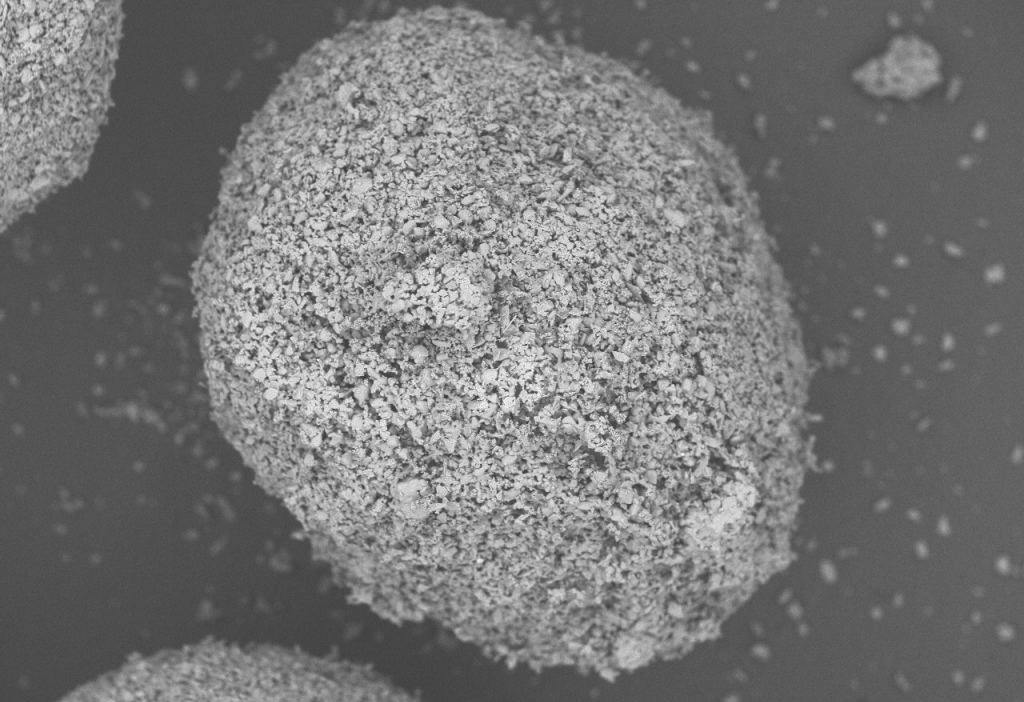
Mo-Sci has been involved with bioglass right from its conception, and with this history, the company is able to offer a unique combination of extensive experience and expertise in glass science, healthcare applications, and product development.
References
- Greenspan D. Bioglass at 50 – A look at Larry Hench’s legacy and bioactive materials. Biomed. Glasses 2019; 5:178–184
- Rahaman M.N., et al. Bioactive glass in tissue engineering. Acta Biomaterialia 2011;7:2355 2373
- Hench L.L. The story of Bioglass, J. Mater. Sci: Mater. Med., 2016, 17, 967-978
- Hench L.L. et al. Bonding mechanisms at the interface of ceramic prosthetic materials. Journal of Biomedical Materials Research Volume 5, Issue 6 p. 117-141
- Jones J.R. Introduction – the discovery of Bioglass. Chapter 12.1 Bioceramics and their Clinical Applications, 2008 edited by Kokubo T. Woodhead Publishing
- Wilson J., et al. Toxicology and biocompatibility of bioglasses. Journal of Biomedical Materials Research Volume 15, Issue 6 p. 805-817
- Kaur G., et al. Mechanical properties of bioactive glasses, ceramics, glass-ceramics and composites: State-of-the-art review and future challenges. Materials Science and Engineering: C Volume 104, November 2019, 109895
- Jones J. Reprint of: Review of bioactive glass: From Hench to hybrids. Acta Biomaterialia Volume 23, Supplement, 1 September 2015, Pages S53-S82
- Hoppe A., et al. In vitro reactivity of Sr-containing bioactive glass (type 1393) nanoparticles. J. Non-Cryst. Solids, 2014, 387, 41-46
- S53P4 bioactive glass in healthcare – International Year of Glass. 2022. S53P4 bioactive glass in healthcare – International Year of Glass – Bonalive
- Brown R.F. et al, Growth and differentiation of osteoblastic cells on 13-93 bioactive glass fibers and scaffolds. Acta Biomater. 2008 Mar;4(2):387-96
- Lopez-Esteban S, et al. Bioactive glass coatings for orthopedic metallic implants. Journal of the European Ceramic Society 2003;23:2921–2930
- Pugely AJ, et al. Influence of 45S5 Bioactive Glass in A Standard Calcium Phosphate Collagen Bone Graft Substitute on the Posterolateral Fusion of Rabbit Spine. Iowa Orthop J. 2017; 37: 193–198.
- Khvostenko D., et al. Bioactive glass fillers reduce bacterial penetration into marginal gaps for composite restorations. Dental Materials Volume 32, Issue 1, January 2016, Pages 73-81
- Islam M.T., et al. Bioactive calcium phosphate–based glasses and ceramics and their biomedical applications: A review. Journal of Tissue Engineering Volume 8, January-December 2017. https://doi.org/10.1177/2041731417719170
- Bioactive glass. Mo-Sci Bioactive Glass – Mo-Sci Mo-Sci Corporation